The 3He Analyzer on the SESAME Beamline
∗ This project is supported by the U.S. Department of Energy
• Introduction
Nuclear spin polarized 3He gas has applications in a diverse range of scientific experiments, including Magnetic Resonance Imaging (MRI)[15], spin-polarized targets for nuclear and high energy physics[11], studies of fundamental interactions[4] and and neutron spin filters [6],[12]. In this case, we use a continuously in situ polarized system[13],[14] as neutron spin filter on the SESAME beamline on the Low Energy Neutron Source (LENS).
The reason polarized 3He can be used as a neutron spin filter is that polarized 3He gas can absorb neutrons through the following inelastic reaction,
n + 3He → 4He∗ → 3He + p
When the neutron spin and the 3He spin are anti-parallel, they feed a broad(270 keV [9]) unbounded excited state of 4He*. The cross section of this process σ+ ≈ 3000[barn]⋅λ is strongly spin dependent and linearly wavelength dependent, following the well-known “1/v” law. When the neutron spin and 3He spin are parallel, the cross section σ- ≈ 5[barn] comes from potential scattering and is small compared to σ+. These properties make polarized 3He gas a good means to polarize/analyze a neutron beam. Centered to this is achieving high 3He polarization.
Compared to other neutron spin analysis methods, 3He has some unique advantages:
1. 3He has a large solid angle for acceptance of scattered/reflected neutrons.
2. 3He operates in a broad neutron wavelength range, which can be tuned by choosing the cell pressure length product.
3. 3He's polarization can be easily flipped by Adiabatic Fast Passage (AFP) technique, thereby combining the function of a neutron polarizer/analyzer and a neutron spin flipper into one device.
4. 3He allows to decouple polarization analyzing from neutron optics because this is a pure absorption based method.
Its disadvantage includes the fact that 3He polarization is time dependent. Continuously pumping in situ can reduce this effect. Our system for doing this is described below.
• Continous pumping 3He Analyzer on the SESAME beamline
The 3He analyzer on SESAME uses Spin Exchange Optical Pumping (SEOP)[10] to polarize 3He. The start point is a diode laser with ~30W CW output (narrowed to 0.24 nm by a Volume Bragg Grating (VBG)), whose spectrum is centered at the RbD1 absorption line (794.7 nm) to pump Rb. Then the angular momentum will be transferred to 3He nucleus through the hyperfine interaction between the Rb electron and 3He nucleus. A polarizer cube is used to split the laser beam into two linearly polarized beams (~ 2:1 ratio). Two quarter-wave plates are used to turn the linearly polarized beams into circularly polarized beams. Finally both of those two circularly polarized light beams are reflected into the oven onto the 3He cell. The oven is kept at 170C° to produce Rb vapor.
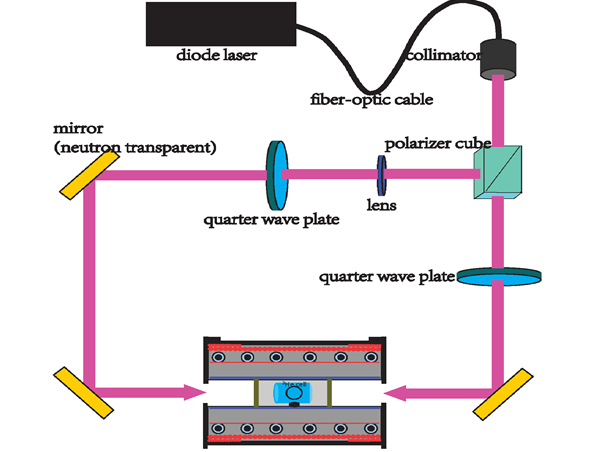
3He Analyzer Device Setup
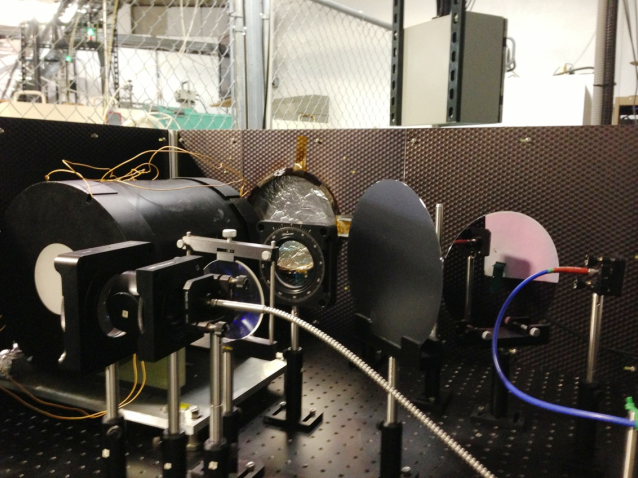
The 3He cell(Mars) is made at NIST[12]. It is a 8cm(length) × 6cm(ID) cylindrical cell with flat windows(0.8cm). The 3He cell is placed in a shielded solenoid which provides uniform magnetic field with field gradient ΔB/B < 3 ×10-4cm-1. In this magnetic field, the T1 was measured to be ~ 100 hours. To monitor the polarization of 3He, both (Free Induction Decay)FID NMR (relative) and Electron Paramagnetic Resonance(EPR)(absolute) techniques are used[14]
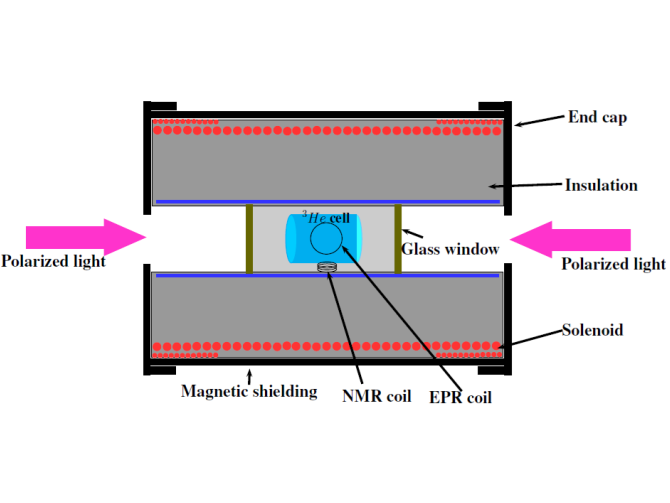
Schematic diagram of the shielded solenoid
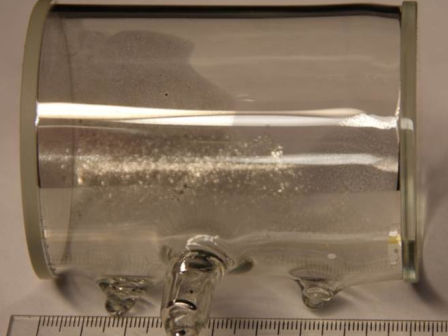
<< Back to Top
• Test Experiment Results
A series of measurements of the 3He polarization using EPR were recently conducted on the SESAME beamline. Showing on right is a plot of 3He polarization measured in the first two test cycle on SESAME beamline.
As can be seen, the 3He suffered from a certain variation in polarization (calculated as 72.6 ± 3.0% and 73.8 ± 3%). The ~3% variation in 3He polarization can introduce a systematic error of neutron polarization measurement on the level of 2%-3% at typical wavelength. One main source of this variation in polarization is the fluctuation of the laser spectrum lineshape. Below is a plot of the laser spectrum lineshape measured off-line. It is clear that during the course of measurement, the lineshape shifts and so is not stable.
To reduce this fluctuation in laser spectrum lineshape, a feedback mechanism was added to the system. The idea is to tune the peak ratio in the laser spectrum to be 1 by adjusting the laser current. A comparison of the peak ratio with (plot (b)) and without (plot (a)) feedback is shown below.

After applying feedback mechanism, two more measurements of polarization were conducted. The polarization is shown below. It can be seen that the polarization is more stable (calculated as 73.4 ± 2.3% and 71.9 ± 1.8%).
Based on this smaller variation, the analyzing power is calculated as well as the difference in analyzing power for the extremes in 3He polarization (maximum and minimum 3He polarization in Cycle 3). This is also shown below. The analyzing power difference is now smaller than 1.5%. So the error in neutron polarization measurement caused by 3He analyzer is generally small.
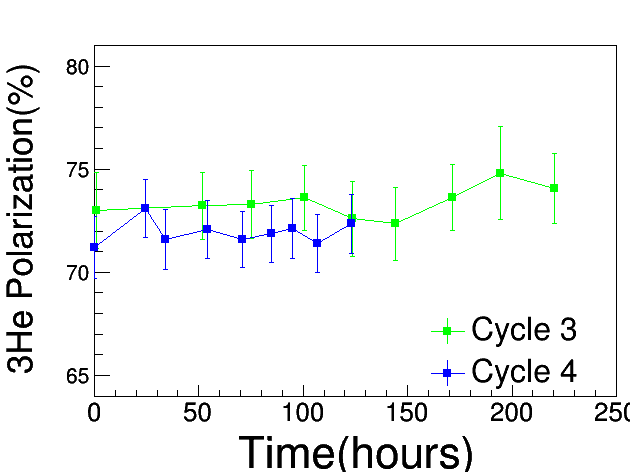
3He polarization vs. time in the last test cycles
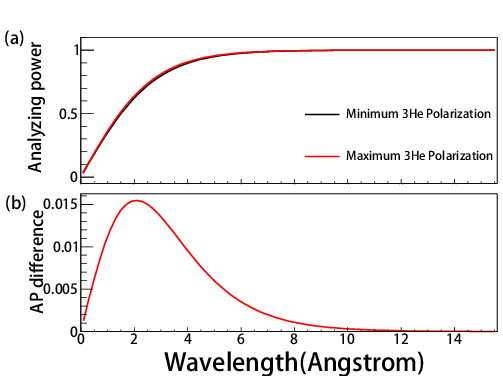
References:
1. Biological magnetic resonance imaging using laser-polarized 129Xe; M.S. Albert, et al. Nature, 370, 199-201, (1994).
2. Analysis of spin-echo small-angle neutron scattering measurements; R. Anderson, et al.; J. Appl. Cryst., 41, 868-885, (2008).
3. Limits to the Polarization for Spin-Exchange Optical Pumping of 3He; E. Babcock, et al.; Phys. Rev. Lett., 96, 083003, (2006).
4. Limit on Lorentz and CPT Violation of the Neutron Using a Two-Species Noble-Gas Maser; D. Bear, et al.; Phys. Rev. Lett., 85, 5038-5041. (2000).
5. Polarized 3He analyzers for neutron reflectometry; W. C. Chen, et al.; Physica B., 335, 196-200, (2003).
6. Test of 3He-based neutron polarizers at NIST; G. Jones, et al.; Instrm. and Methods A, 440, 772-776, (2000).
7. Slow Neutron Cross Sections for 3He, B, and Au; J. Als-Nielsen, et al.; Phys. Rev., 133, B925, (1964).
8. Accurate 3He polarimetry using the Rb Zeeman frequency shift due to the Rb - 3He spin-exchange collisions; M.V. Romalis, et al.; Phys. Rev. A, 58, 3004-3011, (1998).
9. R. Surkau, et al.; Nucl. Instr. and Meth. in Phys. Res. A, 384, 444-450 (1997).
10. Spin-exchange optical pumping of noble-gas nuclei ; T.G. Walker, et al.; Rev. Mod. Phys., 69, 629-642, (1997).
11. Transverse Asymmetry AT' from the Quasielastic 3He→(e→,e') Process and the Neutron Magnetic Form Factor; W. Xu, et al.; Physica B, 356, 96-102, (2005).
12. Polarized 3He image spin filters in neutron scattering; T. R. Gentile, et al.; Phys. Rev. Lett., 85, 2900-2904, (2000).
13. Continuously operating compact image 3He-based neutron spin filter; G. Jones, et al.; . Physica B., 356 86-90, (2005).
14. Continuously operating compact image 3He-based neutron spin filter; G. Jones, et al.; . Physica B., 385 1131-1133, (2006).
15. Take a breath of polarized noble gas; E. W. Otten; . Euro. Phys. News, 35 16-20,(2004).
<< Back to Top